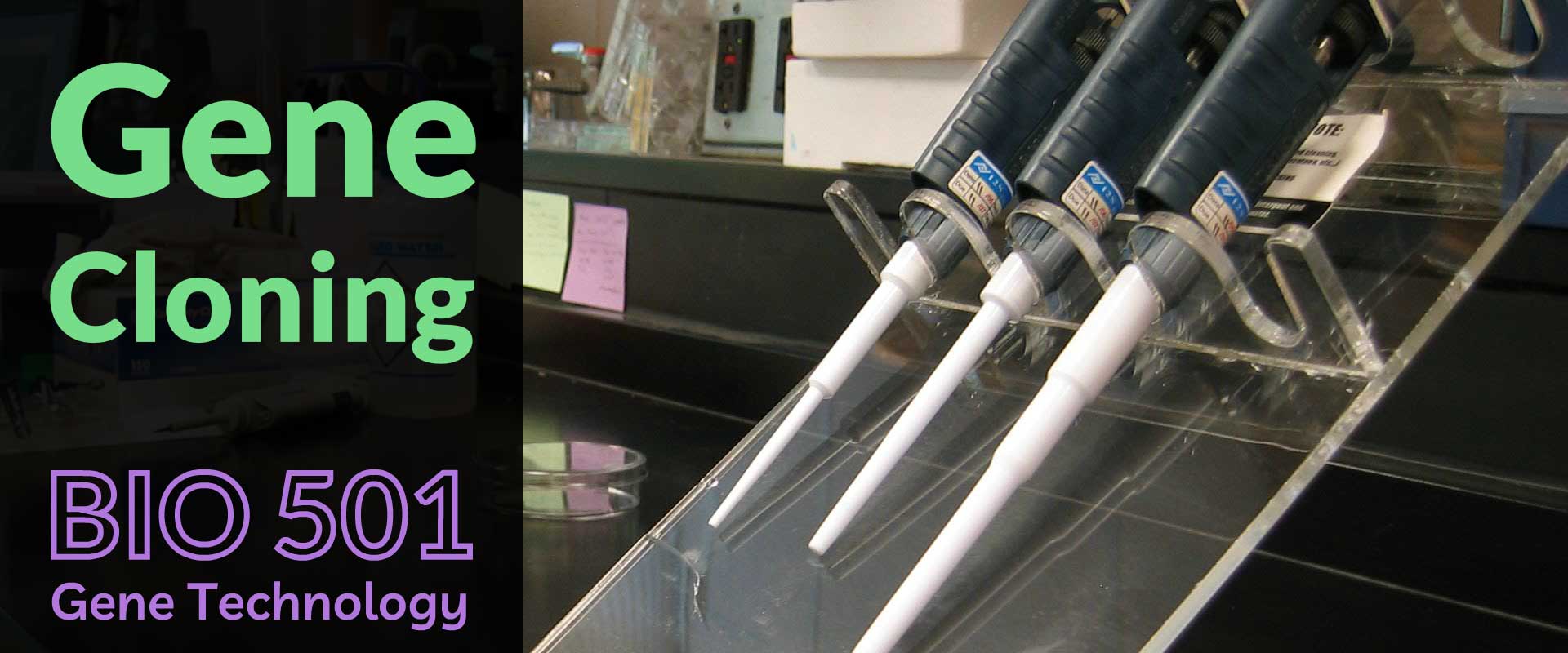
Ultimate Guide to Gene Cloning
Definition of Gene Cloning
Gene cloning is a process of isolation of a gene of interest from the entire pool of genes present in an organism.
Isolated gene is inserted into a bacterial plasmid where it can be easily amplified and studied.
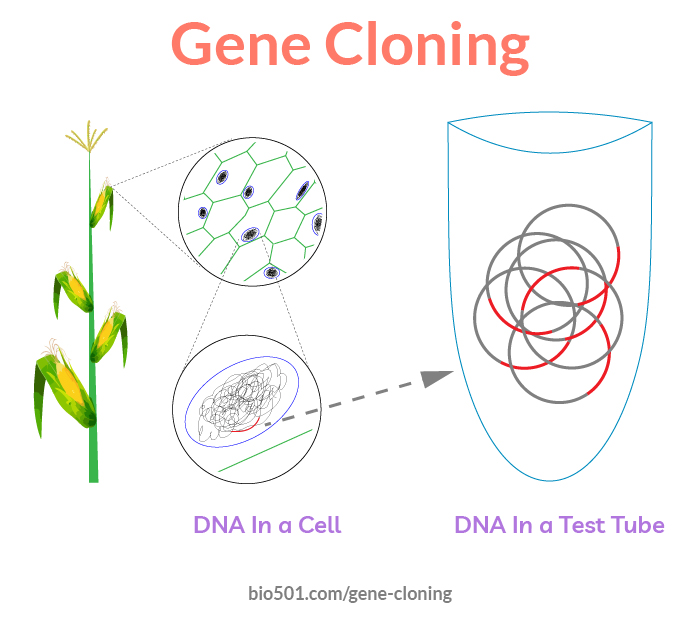
In traditional genetics, a gene is a unit of heredity. It is “something” that is passed from a parent to an offspring, making parents and offsprings look alike.
In modern genetics, a gene is a sequence of DNA. After doing genome sequencing, we can run computer algorithms that predict which DNA regions carry meaningful information and are likely to be genes.
Both traditional genes, and molecular genes, are virtual. They exist on paper and on computer screens. In order to study gene function, or to use a gene in biotechnology, we need to have it in a test tube as a DNA molecule.
Isolating gene DNA from an organism is gene cloning.
Steps of Gene Cloning
The process of gene cloning is divided into several steps, each step representing a separate lab procedure.
Preparation of DNA with gene of interest:
- DNA extraction from cells
- Preparation of complimentary DNA, or cDNA
- Amplification of gene of interest by polymerase chain reaction, or PCR
Creation of recombinant plasmids:
- Cutting of plasmid DNA with restriction enzyme
- Ligation, or joining plasmid and insert DNA
- Transformation of ligated plasmid inside bacteria
- Selection of bacterial colonies

Preparation of DNA with Gene of Interest
Gene cloning from total genomic DNA extract
The simplest way to obtain DNA for gene cloning is to extract genomic DNA from an organism and to cut it with a restriction enzyme.
Genomic DNA extraction is done by following a lab protocol that involves breaking down cells and cleaning up DNA from cell debris.
Restriction enzymes are lab reagents that cut DNA at specific sites, usually six nucleotides long. For example, restriction enzyme BamHI recognizes and cuts DNA sequence GGATCC. Statistically, BamHI recognition sequence happens every 4,096 nucleotides. If we add BamHI to a test tube with genomic DNA extract, it will cut DNA into fragments about 4,000 base-pairs long.
There are three problems with trying to clone a gene directly from genomic DNA:
- In eukaryotic cells, which include yeast, plants, and animals, a gene is broken into coding regions called exons, and non-coding regions called introns. A cell has a dedicated mechanism that cuts out introns and splices introns together. But when we try to clone a gene directly from genomic DNA, we get both exons and introns. In prokaryotes, which include all bacteria, there are no introns, so this problem does not exist when trying to clone a bacterial gene.
- Cutting DNA with a restriction enzyme is a random process that is not selective for a particular gene. With this approach we end up creating a collection of random pieces of genomic DNA. Such collection is called genomic library.
- DNA fragment with our gene of interest will be one among thousands of other fragments created by restriction enzyme.

Gene cloning from cDNA
The central dogma of molecular biology states that genetic information flows from DNA to RNA to protein. Thus genes that are actively transcribed and translated into proteins will have a pool of corresponding messenger RNA molecules floating in cell cytoplasm.
The mRNA pool is already spliced, meaning that all introns have been removed from it. This RNA pool is also representative of genes that are active within a particular cell type.
To clone a gene from mRNA we need to reverse transcribe it, meaning convert it back to DNA.
Reverse transcription is achieved with an enzyme called reverse transcriptase, which is kept as a common reagent in a molecular biology lab. The product of reverse transcriptase activity is called complementary DNA, or cDNA.
Because cDNA fragments are relatively small, they can be cloned into a bacterial plasmid without further cutting by restriction enzymes.
The cDNA approach solves the problem of introns, but it still leaves us with a pool of genes instead of the gene of interest that we are after.
Selective gene amplification by PCR
Polymerase Chain Reaction, or PCR, allows to selectively amplify gene of interest from a pool of cDNA molecules.
During a PCR cycle, a gene of interest is selectively recognized by a pair of artificial DNA primers, and then duplicated by a DNA polymerase. After 30 cycles, a test tube that contained only one copy of a gene, will contain 2 to the power of 30 copies. 2 to the power of 30 is slightly more than a billion.
In order for PCR to work, all we need to know is DNA sequence of the gene of interest, so we can design primers that recognize it among other DNA molecules. In many cases DNA sequence of a gene is known from genome sequencing project or from sequences of similar genes in other organisms.
Artificial gene synthesis
Latest advances in technology allow for genes to be artificially synthesized in a dedicated laboratory. If a gene that you want to work with is under 1000 base pairs long, you can order it from a gene synthesis company.
Artificial gene synthesis saves the headache of extracting mRNA, creating cDNA, and running PCRs.
Creation of Recombinant Plasmids

Bacterial plasmids used for gene cloning
Bacterial plasmid is a circular piece of DNA that can autonomously replicate inside a bacterial cell.
Bacterial plasmids are some of the most useful molecular biology tools, because they can be extracted from bacteria, modified in a million different ways, and then inserted back into bacteria for amplification.
Bacterial plasmids used for gene cloning contain four key features.
- First feature is origin of replication, which allows for plasmid to be replicated inside bacteria.
- Second feature is an antibiotic resistance gene, which is used to selectively kill bacterial cells that do not contain the plasmid, and keep only those that do.
- Third is a multiple cloning site, which can be cleaved by several restriction enzymes.
- And the fourth common feature of plasmids used for gene cloning is LacZ, a beta-galactosidase gene used for blue-white colony screening.
Restriction digest of plasmid and insert DNA
So far we know that a plasmid is a circular piece of DNA, that it contains a multiple cloning site with recognition sequences for restriction enzymes, and that restriction enzymes cut DNA at their recognition sites.
Cutting a plasmid at a multiple cloning site with restriction enzyme opens up the circular molecule and allows for a new gene to be inserted into it.
Insert DNA is also treated with the same restriction enzyme, so the ends of the insert match the ends of the plasmid.
DNA ligation
DNA ligation is the process of stitching insert DNA and plasmid DNA together.
It is done by mixing two sets of DNA molecules in one test tube, and adding another enzyme, called DNA ligase. DNA ligase does the work of joining DNA ends.
The outcome of ligation is the gene of interest integrated into the plasmid vector.
Such fusion is also called recombinant plasmid, “recombinant” meaning that it has been created from DNA pieces that came from unrelated sources.
Bacterial transformation
The recombinant plasmid is then reintroduced back into bacterial cells for propagation and replication.
In molecular biology, introducing new DNA into an organism is called transformation. Bacterial transformation is done by either electric shock – electroporation, or by heat shock, when mixture of bacteria and recombinant plasmid DNA is transferred from ice to a water bath kept at 42 oC. In both cases bacterial membrane structure loosens up, and DNA moves into bacterial cells through membrane pores.
After the transformation, bacterial cells are plated onto a Petri dish with growth medium, where they can multiply and create colonies. Each colony represents a progeny of one individual cell.
Because we only want to keep cells that have taken up recombinant plasmids, growth medium contains an antibiotic, such as ampicillin. Only the cells that acquired ampicillin resistance with introduced plasmid can grow and form colonies on such medium.
Blue-white colony screening
If you were to think think again about DNA ligation, you might realise that in addition to recombinant plasmids, DNA ligase could create other DNA fusions from components present in a test tube.
For example, it can close insert DNA onto itself, or it can stitch several inserts together, or even several plasmids together. Luckily those undesired fusions can’t propage inside a bacterial cell the way a plasmid does.
A byproduct of DNA ligation that does create a problem is vector plasmid that has closed onto itself. It can be introduced inside bacterial cells during transformation. Resulting cells can also grow and form colonies.
Blue-white colony screening has been developed to distinguish between colonies that contain recombinant plasmids, and those that contain plasmids without inserts.
In order to do blue-white colony screening we need to use a plasmid vector that contains beta-galactosidase gene. Bacteria containing such plasmid are grown on a Petri dish with x-gal, a colourless sugar. Beta-galactosidase converts x-gal into a blue metabolite, so their colonies turn blue.
In a recombinant version of the same plasmid, a gene of interest is inserted inside of beta-galactosidase gene, thus disrupting its function. So cells containing recombinant plasmid can not metabolize x-gal and remain white.
By simply looking at the Petri dish, we can distinguish among colonies that have recombinant plasmid, and those that don’t.

Outcome of Gene Cloning
Gene cloning starts with an idea that somewhere hidden in the DNA of an organism there is a small DNA fragment that could be doing something valuable.
During the process of gene cloning we track down that fragment and capture it into a plasmid vector.
Once inside the plasmid vector, we can easily manipulate and study gene of interest by doing DNA sequencing, creating new gene fusions, and reintroducing those back into organisms to see how they behave.
Applications of Gene Cloning
Studying gene function
Gene cloning has been initially developed as a method for gene discovery and for studying gene function.
Every one of tens of thousands of gene studies documented in the National Library of Medicine began with cloning of a gene.
Research on the most critical human diseases genes, such as P53 tumor antigen, TNF-alpha tumor necrosis factor, and BRCA1 tumor suppressor gene, have taken off after those genes were cloned.

Plant biotechnology
Biotech crops, such as Bt corn and Roundup Ready canola, have been created by cloning genes with desired properties and then reintroducing those genes back into crop plants.
Bt corn takes advantage of cry genes cloned from soil bacterium Bacillus thuringiensis.
Cry genes produce crystal proteins that are lethal to certain insect species, but are harmless to other animals and humans. Cloning cry genes and reintroducing them into corn created crop varieties that are resistant to pests. Such corn varieties produce higher yields and no longer require regular application of pesticides.
Roundup Ready canola contains two genes that make it resistant to commonly used herbicide Roundup.
First is EPSPS, a gene cloned from Agrobacterium strain CP4. EPSPS gives plants high tolerance to Roundup.
Second gene is glyphosate oxidase gene from bacterium Ochrobactrum anthropi. Glyphosate oxidase breaks down Roundup within the plant.
Roundup Ready canola solves the problem of weed control.
Prior to its introduction, weeds were controlled by repeatedly plowing the soil. Plowing costs farmers money in tractor diesel fuel, it creates environmental pollution, and leads to rapid soil erosion. With Roundup Ready canola weeds are controlled by spraying farm fields with Roundup. Roundup kills all the weeds and leaves resistant crops intact.
Production of recombinant proteins
Production of recombinant insulin is a classic example of application of gene cloning in biotechnology.
From 1920-s insulin derived from cow and pig pancreas was used to treat patients with diabetes. Insulin derived from animals was causing allergic reactions, and its supply was declining, while the number of patients with diabetes was going up.
Humulin was the first human recombinant insulin approved by the FDA for the treatment of diabetes.
Humulin was developed by cloning the human insulin gene, and then by using E. coli to produce protein in fermentation tanks.
Because recombinant insulin is based on the human gene, it does not cause allergic reaction.
And, as you can imagine, it’s supply is limitless.